In touch with dark matter at the Large Hadron Collider: possible or preposterous?
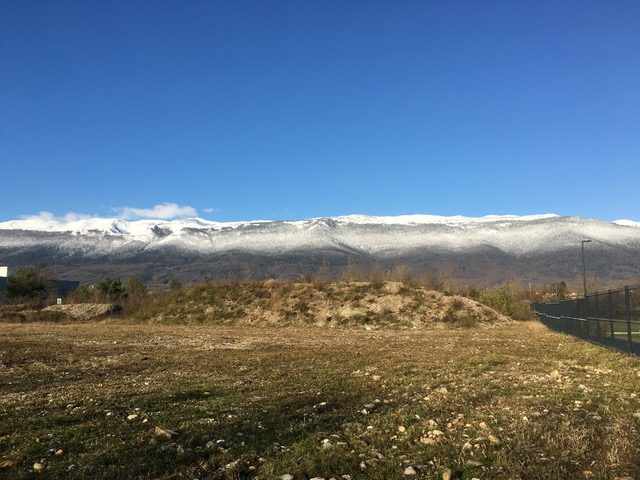
A new day dawns at the Swiss-French border
It is 4am in a small village close to the Swiss and French border. The sound of a cock crowing rings through the air. However, this sound does not signal the dawn of a new day but the injection of a new beam in the world’s most powerful particle accelerator. I am in the ATLAS control room. Instead of a rural scenery, the room is packed with computer monitors, projectors, and loudspeakers. The audio signal tells us, the shift crew, that the start of a new run is imminent and we have to get the detectors ready for another round of data-taking.
The ATLAS detector is a multi-purpose particle detector and perhaps the biggest 100 Megapixel camera ever built. It was designed by thousands of particle physicists and engineers to record the debris created in the interactions of the two colliding proton beams.
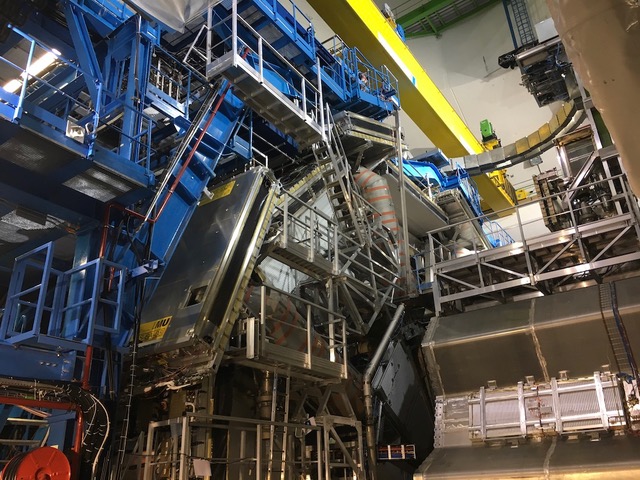
After my shift, on my way back to the CERN hostel, I look up to the clear night sky above the Jura mountains. These moments make me remember the questions which my five-year-old self used to ask: “What is the Universe made of?”
Over the last century, a fairly compelling answer to this question has evolved. The immodestly titled “Standard Model of Particle Physics” enumerates the elementary building blocks of matter. I like to compare the particles in the Standard Model to Lego blocks. Much like the creations of children playing with these building blocks, everything around us is made up of three different kinds of elementary particles and their mutual interactions.
Below my feet, the Large Hadron collider continues its operation, providing data which to date confirms this scientific narrative. A story too good to be true? Perhaps, as there is a catch.
Dark matter in our universe
The particles in the Standard Model make up only a fraction of the matter content in the Universe. According to astrophysical observations, there is more matter in the Universe than just the visible matter which we experience in everyday life. Even the matter provided by all the planets, stars, galaxies and even the enormous amounts of intergalactic dust is not enough: the major fraction of the Universe is made up from something which cannot be observed directly in astronomy and which we don’t understand well – so-called “dark matter”.
The only properties of dark matter we are certain about are its mass and its lack of observable interactions. These properties are established by the gravitational pull of dark matter on other (visible) astrophysical objects.
Although most of our current understanding of dark matter originates from indirect observations, remarkably, dark matter particles could be produced at the Large Hadron Collider. In my PhD at the Max-Planck-Institute for Physics, I investigated data collected at the Large Hadron Collider with ATLAS in the years 2015 – 2018 for traces of such dark matter particles.
At first glance, such an endeavour must seem preposterous: even if dark matter particles are produced at the Large Hadron Collider, they would still be invisible to the detectors. If we picture the ATLAS data as photographs of the collisions, then in searches for these elusive particles, we are blindfolded.
We need a clever way to detect the dark matter particles in a different way. Do you recall playing “blind man’s buff” at a child birthday party? When being blindfolded, you need to rely on your sense of touch for identifying your surroundings. In dark matter searches, a similar strategy is pursued: although dark matter particles are invisible, their potential effect on other objects is not. When other particles are produced in the same proton collision, they recoil against the dark matter particles. Therefore, we can use these other particles as a “cane” to search for dark matter.
The key to the invisible universe: missing transverse momentum
Of course, this is a simplified picture. The rigorous justification for this type of dark matter searches comes from one of the pillars of physics which can be traced back to Isaac Newton: momentum conservation.
Let us examine a typical collision of two proton beams: Prior to the collisions, the protons in the Large Hadron Collider happily fly along the first beam pipe, accelerated close to the speed of light and unaware of their eventual and terrible fate: in the centre of the ATLAS detector the proton beam intersects with a second beam, resulting in spectacular collisions of protons being smashed together. Unlike in car crashes, not only the debris of the protons shoots through the layers of detector material. The energy in the proton collisions is so large that even pairs of new particles can be created.
What about the momentum balance prior and after the collision? Prior to the collision, the total momentum is fully aligned with the beam pipe. All protons circle in the beam, there is no movement in the plane perpendicular to the beam pipe. The net momentum in the so-called transverse plane is zero.
By the means of momentum conservation, this must also hold after the collision took place. If a pair of particles is created in the collision, the particles must fly in exactly opposite directions in the transverse plane to allow their momentum components to cancel out. If the net momentum of all particles created in the collision is not zero, then some invisible particle must have escaped the detectors.
Therefore, the associated observable is called “missing transverse momentum” – the missing momentum component in the plane transverse to the beam pipe.
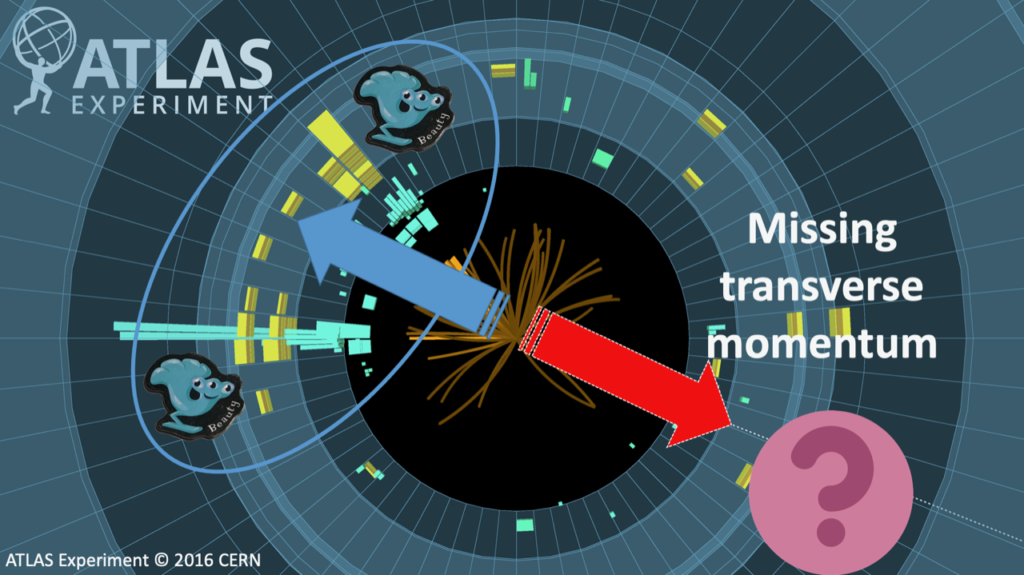
Finally! This observable allows us to infer the presence of dark matter particles which might be produced in the collision. The missing transverse momentum is at the heart of dark matter searches performed with ATLAS data. The other defining property of these searches is the type of other particle which is recoiling. I investigated searches for dark matter produced with W/Z bosons, Higgs bosons and even pairs of W/Z bosons.
Is it that easy? Just searching for collision events with large missing transverse momentum and you can claim to have discovered dark matter?
Experimental challenge: invisible background
Of course, the answer is no. Not only do these searches need to account for every possible way, in which performance effects of the detectors or some particle reconstruction inefficiencies could lead towards fake missing transverse momentum: there are even particles described by the Standard Model which are invisible to the detector. These particles are called neutrinos and are sometimes referred to as “ghost particles” because of their extremely feeble interaction with other particles. By the time you read the article, a large numbers of solar neutrinos will have passed through your body and continue on their way without leaving any trace. Similarly, they produce the same signature as dark matter. Is all hope lost in detecting dark matter among background processes?
Fortunately, theoretical physicists have calculated how likely such background processes occur. In practice, the observed collision events are categorised by the missing transverse momentum and displayed as a histogram. A histograms shows how many events are associated to a certain range in missing transverse momentum. Similarly, the predictions from the theorists for the contribution of neutrino background processes and the potentially additional contribution from dark matter particles is categorised. In the end, the data are compared with the prediction to decide what is more likely: just the background hypothesis, or perhaps the dark matter hypothesis?
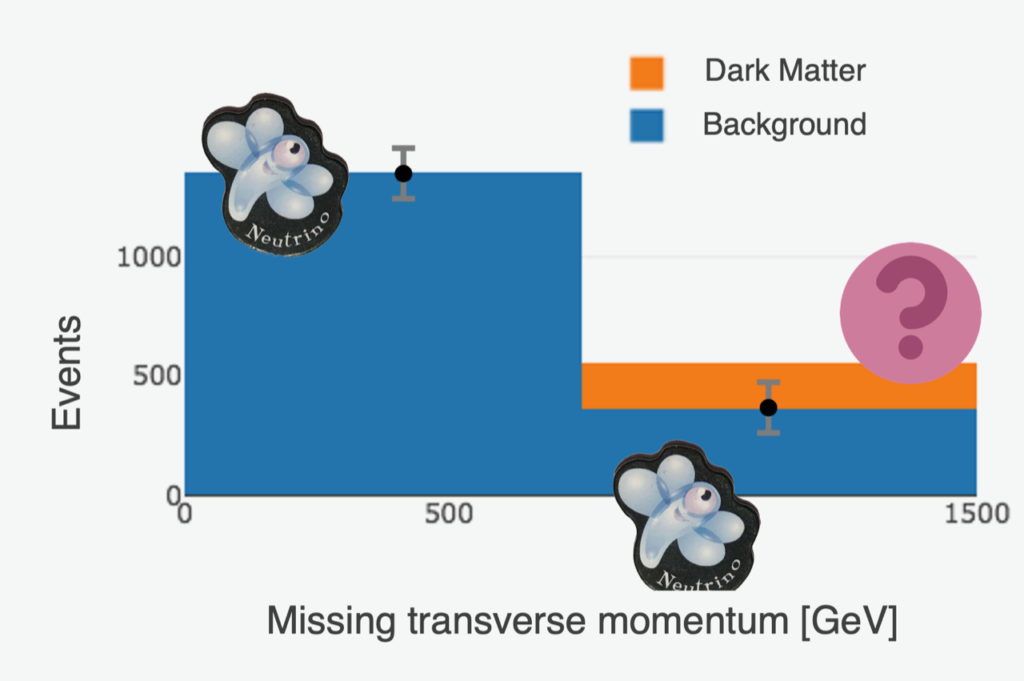
Search for dark matter in events with Higgs bosons
Now that we have established how to search for dark matter, let us look at the results of the real thing. Below, you can see another histogram, which shows the missing transverse momentum distribution measured in a search for dark matter produced in association with a Higgs boson. Of course, the distribution looks more complicated than the example above (e.g. there is a more detailed list of potential background processes and the prediction for dark matter particles is not stacked but overlaid), but the general structure is the same.
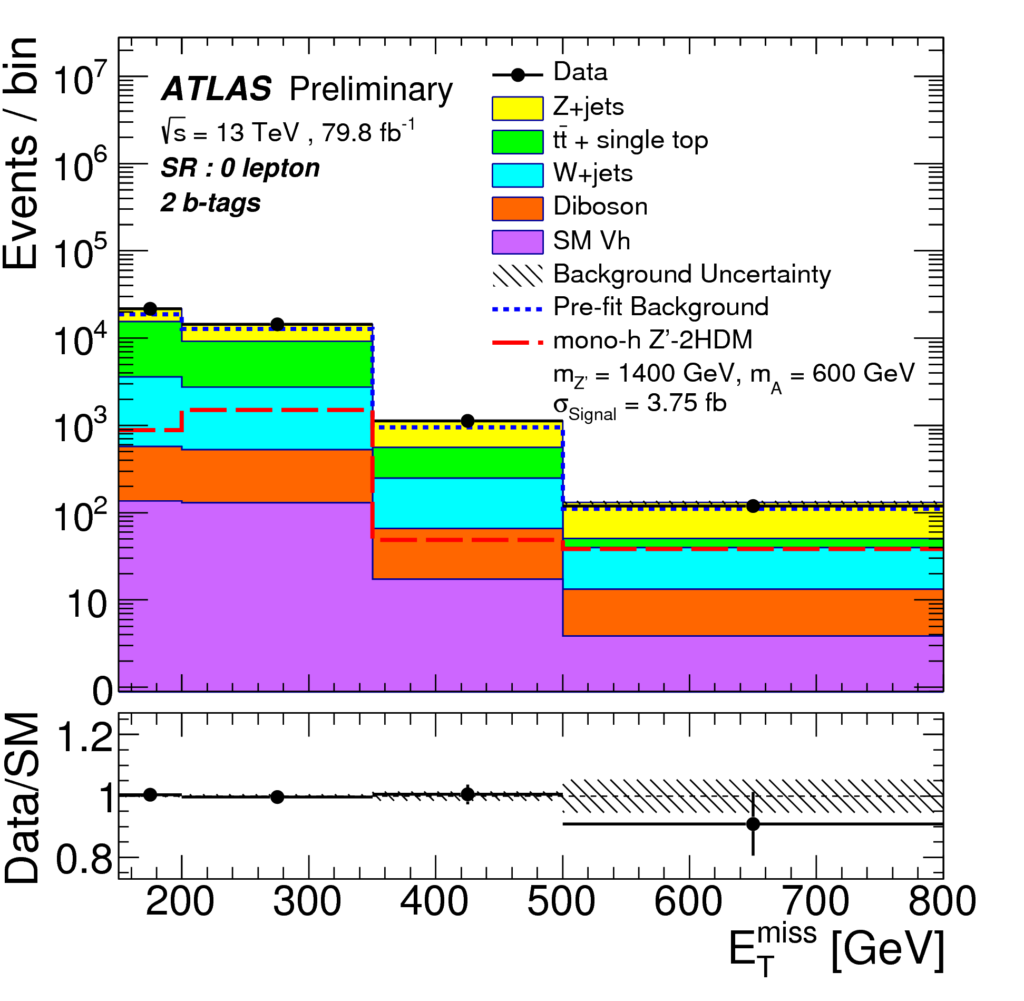
The message from the experiment is clear: all data points agree perfectly with the background from the Standard Model. There is no sign for events occurring with missing transverse momentum which could be associated to dark matter particles created at the Large Hadron Collider.
Does that mean that all the time and effort in the ATLAS control room, in designing algorithms for data analysis, countless hours of statistical analysis, all has been in vain? The opposite is the case! These null-results from the Large Hadron Colliders are vital information for theoretical physicists pondering how dark matter could be realised in our Universe. Ultimately, experiment is the final arbiter of a theory in empiric sciences. Therefore, these results challenge models of dark matter particles and help to rule out certain hypotheses, paving the way to a better understanding of the dark matter puzzle.
If you want to read more, check out the ATLAS publication: https://atlas.web.cern.ch/Atlas/GROUPS/PHYSICS/CONFNOTES/ATLAS-CONF-2018-039/
If you understand German, you can have a look at a video explaining this type of dark matter searches at the Large Hadron Collider: